
What is bioelectricity?
Bioelectricity is electricity generated by living things. It is essential for many kinds of signaling in organisms ranging from bacteria to human beings. Bioelectricity provides a mechanism for ultrarapid signaling between cells, using signaling particles readily available in the environment, i.e., charged ions. As their name suggests, proteins named 'ion channels' provide conduits for these charged ions to travel between different spaces - a common example being from the extracellular to intracellular space, or vice versa.
The inside of the cell is separated from the outside of the cell by a hydrophobic barrier formed from a lipid bilayer termed the 'plasma membrane'. Because of the hydrophobicity of their interiors, lipid bilayers are excellent insulators and prevent charged aqueous ions from freely moving between compartments. Ion channels are membrane-spanning proteins that provide a means to control the movement of the charged ions across lipid bilayers, both in terms of which ion species travel and when they travel. This is acheived without energy expenditure, as ions pass through ion channels down an electrochemical gradient, by diffusion. The mechanisms by which this is achieved are some of the most fascinating in biology. How can an ion channel distinguish between similarly charged ions, and even permit potassium ions (K+) to diffuse while disallowing the similarly charged sodium ion (Na+) which is smaller than K+? Recent advances in atomic-level structures of ion channels have revealed the secrets of their 'selectivity filters'. The ability to differentiate between K+ and Na+ is prerequisite for generation of action potentials - arguably the most important unit of signaling in the animal kingdom. In an action potential, an excitable cell, which is normally negatively charged at rest compared to the outside if the cell, first depolarizes (to a positive membrane potential) and then repolarizes (back to a negative membrane potential). The depolarization turns on processes in the cell, a common mechanism being an increase in cytosolic calcium ions (Ca2+). The repolarization returns the cell to its resting state, readying it for another signal.
The cellular depolarization is, in most excitable cells, achieved by an influx of Na+ through voltage-dependent sodium (Nav) channels. The repolarization is almost always achieved by efflux of K+ through voltage-dependent potassium (Kv) channels). Voltage dependence of ion channels is endowed by another miracle of biology - a positively charged domain that spans the lipid bilayer and senses the potential difference between the inside and the exterior of the cell. Nav and Kv voltage sensors are activated by membrane depolarization, opening the channel to permit ion diffusion in response to a change in membrane potential. Another class of channel, named 'pacemaker' or 'hyperpolarization-activated' channel, is activated by hyperpolarization.
Research directions in the Abbott lab
KCNE proteins
In the Abbott lab, we focus primarily on potassium channels. The pore-forming subunits of channels are typically referred to as the α subunits. These proteins contain, in Kv channels, a K+ selectivity filter and ion conduction pathway (collectively referred to as the pore) and a voltage-sensing domain (VSD). Four Kv α subunits coassemble to form a functional unit. Ion channels are however, normally macromolecular complexes made up of both α subunits and other, regulatory (β) subunits in vivo. We study how these complexes function and travel to their site of action in the cell - the plasma membrane - and what happens after they are internalized away from the plasma membrane. In particular, we study how Kv channels are regulated by a class of β or ancillary subunits encoded by the five members of the KCNE gene family. These KCNE proteins (which we also named MinK-related peptides, or MiRPs) are single-transmembrane domain proteins that co-assemble with Kv α subunits and regulate or control their trafficking (anterograde and retrograde), ion conductance and selectivity, gating kinetics (how fast or slow the channels open and close), voltage dependence (how the voltage sensor responds to membrane potential) and regulation by other proteins, lipids, and drugs.
The KCNE proteins appear to be required to generate the diversity of potassium current required for higher animals to function. We suspect that the scope of KCNE regulation of ion channels and other functions in vivo is not yet fully appreciated. Occasional hints suggest KCNE proteins may play other roles in physiology beyond regulating ion channels, or at least connecting ion channel activity to the function of other proteins or processes not currently considered to regulate channel function. Among other research directions, we are pursuing discovery of these exotic roles of KCNE proteins, utilizing techniques as diverse as transcriptomics, yeast two-hybrid, mouse genetics and electrophysiology.
The macromolecular signaling complexes we study in the Abbott lab are exemplified by those containing the KCNQ1 voltage-gated K+ (Kv) channel α subunit and KCNE family β subunits. KCNQ1 is a ubiquitous, multifunctional α subunit, genetically linked to pernicious human disorders including lethal cardiac arrhythmias, gastric cancer, and diabetes [1]. The KCNE β subunits are best known for modifying the functional properties of cardiac Kv channel α subunits including KCNQ1, and for their association with human cardiac arrhythmias including Long QT Syndrome (LQTS), Brugada Syndrome (BrS) and atrial fibrillation (AF) [2,3]. Increasing evidence supports an even broader role for KCNE subunits, encompassing most human tissues, regulation of e.g., pacemaker (HCN) and Ca2+ channels, and also the ability to dictate aspects of channel biology beyond the electrical attributes - including trafficking, α subunit composition of channels, and regulation by drugs, pH, and PIP2 [4-11]. Studies of Kcne gene knockout (Kcne–/–) mice have uncovered crucial extracardiac KCNE activities, and predicted disorders that were subsequently linked to human gene disruption [12-16]. Because the five KCNE genes exhibit non-redundant functions, and are requisite components of many native ion channels, Kcne–/– mice can be used to uncover essential native roles not just for KCNE subunits, but for KCNQ1 and other α subunits they regulate [17-19].
KCNE Genes at the Nexus of Genetic and Environmental Factors in Metabolic Disease and SCD
The majority of previous research into genes linked to sudden cardiac death (SCD) has, quite appropriately, focused on their direct roles in cardiac myocytes. However, most of the 25 genes currently associated with arrhythmia/SCD are expressed in other tissues in addition to the heart. The novel strategy adopted in the Abbott lab, highlighted by recent projects on KCNE2 and KCNE3 [17,19-22], has been to explore both the cardiac and extracardiac effects of gene disruption, and also the interplay between the two, facilitated by a holistic approach including gene-targeted mice and “omics” analyses (Figure 1).
Our holistic research approach began with studies demonstrating that KCNQ1-KCNE2 is required for normal thyroid hormone synthesis and that Kcne2 deletion causes hypothyroidism that is deleterious to cardiac function [19,23]. We next incorporated transcriptomics and computer modeling as part of a systems approach to understanding the global ramifications of disruption of genes linked to human cardiac arrhythmias. This research led to the first description of an entirely extracardiac source of monogenic cardiac arrhythmogenesis (adrenal autoimmune response arising from Kcne3 deletion) [20]. It also led to our discovery that Kcne2 deletion causes diabetes, elevated LDL cholesterol and Ang II, anemia, hyperkalemia and aging-associated LQTS [21,22]. We described how fasting in Kcne2–/– diabetic mice causes hypoglycemia-specific arrhythmias (A/V block, whereas the non-fasted Kcne2-/- mice developed ventricular fibrillation without A/V block) [21] – analogous to elements of human diabetic Dead-in-Bed Syndrome.
Discovery of a Novel Class of Macromolecular Signaling Complexes: 'Chansporters'
We recently discovered a novel class of signaling complexes in which ion channels interact directly with solute transporters (Figure 2). Utilizing global metabolite profiling by mass spectrometry, we revealed that mice lacking the KCNE2 β subunit showed a deficiency in myo-inositol in cerebrospinal fluid (CSF) but not in serum. Increased stress responsiveness and seizure susceptibility in Kcne2–/– mice were alleviated by myo-inositol supplementation. Suspecting a defect in myo-inositol transport in the choroid plexus epithelium, we found that KCNE2 and KCNQ1 co-assemble in the choroid plexus epithelium with SMIT1, the primary Na+-coupled myo-inositol transporter in this tissue [24].
Na+-coupled transport, via membrane-spanning SLC5 family transporter proteins, is crucial for uptake of solutes including ions, sugars and myo-inositol, an important osmolyte and precursor for cell signaling molecules. SMIT1 transports both myo-inositol and the less abundant but equally essential stereoisomer, scyllo-inositol, into cells. Myo-inositol imbalances have been linked to seizure susceptibility and schizophrenia but underlying mechanisms remain elusive and controversial. Myo-inositol also exhibits insulin-mimetic properties and can lower post-prandial blood glucose. Scyllo-inositol is also of great interest because it inhibits of Ab fibril formation and is in clinical trials for treatment of Alzheimer’s disease.
In ongoing and future studies, we will examine possible complexes formed by a wide range of clinically important channels and transporters. We will use a variety of approaches to determine the molecular mechanisms of interaction and function of this novel clas of signaling complexes.
Versatility of KCNE proteins
Initially, KCNE proteins were thought to modulate solely the electrical properties of Kv channels, such as voltage-dependence and conductance. we now know that KCNE proteins are much more versatile, regulating many aspects of Kv channel biology including trafficking, pharmacology (Figure 3) and even α subunit composition (Figure 4) [5-8].



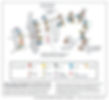
References
1. Abbott, G. W. Biology of the KCNQ1 potassium channel. New Journal of Science 2014, doi:http://dx/doi.org/10.1155/2014/237431 (2014).
2. Crump, S. M. & Abbott, G. W. Arrhythmogenic KCNE gene variants: current knowledge and future challenges. Frontiers in genetics 5, 3, doi:10.3389/fgene.2014.00003 (2014).
3. McCrossan, Z. A. & Abbott, G. W. The MinK-related peptides. Neuropharmacology 47, 787-821, doi:10.1016/j.neuropharm.2004.06.018 (2004).
4. Abbott, G. W. KCNE2 and the K (+) channel: The tail wagging the dog. Channels (Austin) 6 (2012).
5. Kanda, V. A. & Abbott, G. W. KCNE Regulation of K(+) Channel Trafficking - a Sisyphean Task? Frontiers in physiology 3, 231, doi:10.3389/fphys.2012.00231 (2012).
6. Kanda, V. A., Lewis, A., Xu, X. & Abbott, G. W. KCNE1 and KCNE2 inhibit forward trafficking of homomeric N-type voltage-gated potassium channels. Biophysical journal in press (2011).
7. Kanda, V. A., Lewis, A., Xu, X. & Abbott, G. W. KCNE1 and KCNE2 provide a checkpoint governing voltage-gated potassium channel alpha-subunit composition. Biophysical journal 101, 1364-1375, doi:10.1016/j.bpj.2011.08.014 (2011).
8. Kanda, V. A., Purtell, K. & Abbott, G. W. Protein kinase C downregulates I(Ks) by stimulating KCNQ1-KCNE1 potassium channel endocytosis. Heart rhythm : the official journal of the Heart Rhythm Society 8, 1641-1647, doi:10.1016/j.hrthm.2011.04.034 (2011).
9. Nawathe, P. A., Kryukova, Y., Oren, R. V., Milanesi, R., Clancy, C. E., Lu, J. T., Moss, A. J., Difrancesco, D. & Robinson, R. B. An LQTS6 MiRP1 mutation suppresses pacemaker current and is associated with sinus bradycardia. Journal of cardiovascular electrophysiology 24, 1021-1027, doi:10.1111/jce.12163 (2013).
10. Qu, J., Kryukova, Y., Potapova, I. A., Doronin, S. V., Larsen, M., Krishnamurthy, G., Cohen, I. S. & Robinson, R. B. MiRP1 modulates HCN2 channel expression and gating in cardiac myocytes. The Journal of biological chemistry 279, 43497-43502, doi:10.1074/jbc.M405018200 (2004).
11. Liu, W., Deng, J., Wang, G., Zhang, C., Luo, X., Yan, D., Su, Q. & Liu, J. KCNE2 modulates cardiac L-type Ca channel. Journal of molecular and cellular cardiology, doi:10.1016/j.yjmcc.2014.03.013 (2014).
12. Lee, M. P., Ravenel, J. D., Hu, R. J., Lustig, L. R., Tomaselli, G., Berger, R. D., Brandenburg, S. A., Litzi, T. J., Bunton, T. E., Limb, C., Francis, H., Gorelikow, M., Gu, H., Washington, K., Argani, P., Goldenring, J. R., Coffey, R. J. & Feinberg, A. P. Targeted disruption of the Kvlqt1 gene causes deafness and gastric hyperplasia in mice. The Journal of clinical investigation 106, 1447-1455, doi:10.1172/JCI10897 (2000).
13. Roepke, T. K., Anantharam, A., Kirchhoff, P., Busque, S. M., Young, J. B., Geibel, J. P., Lerner, D. J. & Abbott, G. W. The KCNE2 potassium channel ancillary subunit is essential for gastric acid secretion. The Journal of biological chemistry 281, 23740-23747, doi:10.1074/jbc.M604155200 (2006).
14. Roepke, T. K., Purtell, K., King, E. C., La Perle, K. M., Lerner, D. J. & Abbott, G. W. Targeted deletion of Kcne2 causes gastritis cystica profunda and gastric neoplasia. PloS one 5, e11451, doi:10.1371/journal.pone.0011451 (2010).
15. Rice, K. S., Dickson, G., Lane, M., Crawford, J., Chung, S. K., Rees, M. I., Shelling, A. N., Love, D. R. & Skinner, J. R. Elevated serum gastrin levels in Jervell and Lange-Nielsen syndrome: a marker of severe KCNQ1 dysfunction? Heart rhythm : the official journal of the Heart Rhythm Society 8, 551-554, doi:10.1016/j.hrthm.2010.11.039 (2011).
16. Kuwahara, N., Kitazawa, R., Fujiishi, K., Nagai, Y., Haraguchi, R. & Kitazawa, S. Gastric adenocarcinoma arising in gastritis cystica profunda presenting with selective loss of KCNE2 expression. World journal of gastroenterology : WJG 19, 1314-1317, doi:10.3748/wjg.v19.i8.1314 (2013).
17. Abbott, G. W., Tai, K. K., Neverisky, D. L., Hansler, A., Hu, Z., Roepke, T. K., Lerner, D. J., Chen, Q., Liu, L., Zupan, B., Toth, M., Haynes, R., Huang, X., Demirbas, D., Buccafusca, R., Gross, S. S., Kanda, V. A. & Berry, G. T. KCNQ1, KCNE2, and Na+-Coupled Solute Transporters Form Reciprocally Regulating Complexes That Affect Neuronal Excitability. Science signaling 7, ra22, doi:10.1126/scisignal.2005025 (2014).
18. Roepke, T. K., Kanda, V. A., Purtell, K., King, E. C., Lerner, D. J. & Abbott, G. W. KCNE2 forms potassium channels with KCNA3 and KCNQ1 in the choroid plexus epithelium. The FASEB journal : official publication of the Federation of American Societies for Experimental Biology 25, 4264-4273 (2011).
19. Roepke, T. K., King, E. C., Reyna-Neyra, A., Paroder, M., Purtell, K., Koba, W., Fine, E., Lerner, D. J., Carrasco, N. & Abbott, G. W. Kcne2 deletion uncovers its crucial role in thyroid hormone biosynthesis. Nature medicine 15, 1186-1194, doi:10.1038/nm.2029 (2009).
20. Hu, Z., Crump, S. M., Anand, M., Kant, R., Levi, R. & Abbott, G. W. Kcne3 deletion initiates extracardiac arrhythmogenesis in mice. The FASEB journal : official publication of the Federation of American Societies for Experimental Biology 28, 935-945, doi:10.1096/fj.13-241828 (2014).
21 Hu, Z., Kant, R., Anand, M., King, E. C., Krogh-Madsen, T., Christini, D. J. & Abbott, G. W. Kcne2 Deletion Creates a Multisystem Syndrome Predisposing to Sudden Cardiac Death. Circulation. Cardiovascular genetics, doi:10.1161/CIRCGENETICS.113.000315 (2014).
22. Duff, H. J. Beyond doctrine and dogma: one gene--multisystem effects. Circulation. Cardiovascular genetics 7, 4, doi:10.1161/CIRCGENETICS.114.000508 (2014).
23. Purtell, K., Paroder-Belenitsky, M., Reyna-Neyra, A., Nicola, J. P., Koba, W., Fine, E., Carrasco, N. & Abbott, G. W. The KCNQ1-KCNE2 K+ channel is required for adequate thyroid I- uptake. The FASEB journal : official publication of the Federation of American Societies for Experimental Biology, doi:10.1096/fj.12-206110 (2012).
24. Abbott, G. W. et al. KCNQ1, KCNE2, and Na+-Coupled Solute Transporters Form Reciprocally Regulating Complexes That Affect Neuronal Excitability. Science signaling 7, ra22, doi:10.1126/scisignal.2005025 (2014).